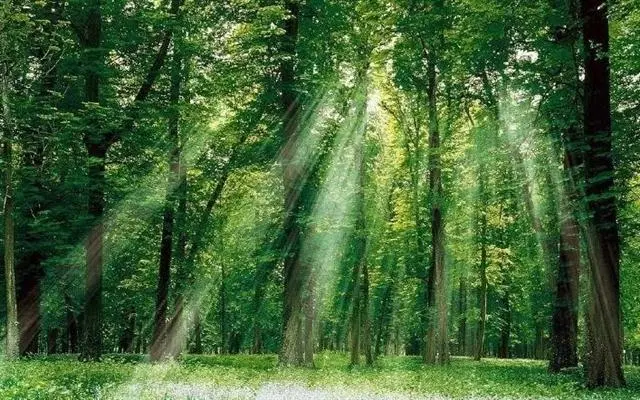
Understanding the Basics of Photosynthesis
Photosynthesis is a fundamental biological process through which plants convert solar energy into chemical energy. This transformation is crucial for the sustenance of nearly all life forms on Earth, as it forms the base of the food chain. Photosynthesis primarily occurs in the chloroplasts of plant cells, where the pigment chlorophyll plays a pivotal role. Chlorophyll absorbs sunlight, which provides the necessary energy to drive the photosynthetic reactions.
During photosynthesis, plants take in carbon dioxide (CO2) from the atmosphere through small openings called stomata. Simultaneously, water (H2O) is absorbed by the roots from the soil. These inputs are transported to the chloroplasts, where the light-dependent reactions of photosynthesis take place. In these reactions, solar energy is harnessed to split water molecules into oxygen (O2), protons, and electrons. The oxygen is released as a by-product, while the protons and electrons are utilized in the formation of adenosine triphosphate (ATP) and nicotinamide adenine dinucleotide phosphate (NADPH), which are crucial energy carriers.
The subsequent stage, known as the Calvin Cycle or light-independent reactions, uses the ATP and NADPH produced to convert carbon dioxide into glucose (C6H12O6). This glucose serves as an essential source of energy and a building block for other organic molecules within the plant. Additionally, it can be stored as starch for later use or transformed into cellulose, which contributes to the structural integrity of the plant.
The significance of photosynthesis extends beyond individual plants to the broader context of agriculture and food production. By understanding and optimizing the process of photosynthesis, farmers and agricultural scientists can enhance crop yields. Effective crop configurations and strategic placement can maximize sunlight capture, ensuring that plants receive adequate energy to perform photosynthesis efficiently. This, in turn, can lead to improved growth, higher productivity, and better resilience against environmental stresses.
The Science Behind Crop Configurations
Understanding the science behind crop configurations is fundamental to optimizing sunlight capture, which directly influences photosynthetic efficiency and crop yield. The arrangement of plants, row spacing, and plant density are critical factors that determine how effectively a crop canopy intercepts light. By strategically configuring these elements, farmers can enhance light interception and, consequently, the overall photosynthetic activity of the plants.
One traditional approach is the rectangular planting system, where crops are sown in parallel rows. This configuration allows for efficient use of machinery but may result in uneven light distribution, especially as plants grow larger and create shade. Adjusting row spacing can mitigate some of these effects. Wider rows can reduce shading but may also lead to lower plant density, affecting total biomass production.
Innovative configurations, such as the equidistant triangle planting system, offer a more uniform distribution of plants. This method maximizes light interception by ensuring that each plant has equal access to sunlight, thereby reducing intra-row shading. Research has shown that such configurations can significantly improve photosynthetic efficiency and increase crop yields.
Plant density also plays a critical role. Higher plant densities can lead to increased competition for light, nutrients, and water, potentially reducing individual plant growth. However, at optimal densities, crops can form a canopy that captures sunlight more effectively, enhancing overall biomass production. Conversely, lower densities may not fully utilize available light, resulting in wasted solar energy.
Examples of these principles in practice include the “skip-row” method, where certain rows are left unplanted to increase light penetration to lower leaves, and the “double-row” system, which involves planting two closely spaced rows with a larger gap between pairs. Both methods aim to balance plant density and light distribution for optimal growth.
Scientific principles such as the Beer-Lambert law, which describes light attenuation through a medium, and the concept of Leaf Area Index (LAI), which measures the leaf surface area per unit ground area, underpin these configurations. By understanding and applying these principles, farmers can design crop configurations that maximize sunlight capture, leading to higher photosynthetic efficiency and improved crop yields.
Practical Applications and Techniques
Implementing sunshine strategies in agricultural practices requires a blend of traditional methods and modern technologies. By optimizing crop configurations to capture more sunlight, farmers can significantly enhance their yields and overall efficiency. One practical technique involves the strategic alignment of crop rows. For instance, east-west row orientation maximizes sunlight capture for crops during the morning and late afternoon, while north-south orientation can be beneficial in regions with intense midday sun, ensuring even light distribution.
Case studies provide valuable insights into these methods. Consider a Midwest corn farm that adopted a modified row spacing technique. By increasing the distance between rows, the farmer ensured that each plant received adequate sunlight, resulting in a 15% increase in yield. Another example is a vineyard in California that utilized reflective mulches to direct more sunlight to the grapevines, enhancing grape quality and production.
Step-by-step guides can help farmers adapt these strategies to various crops and conditions. Start by assessing the specific light requirements of the crops and the geographical orientation of the fields. Next, experiment with different row configurations and spacing to find the most effective pattern for sunlight capture. Regular monitoring and adjustments based on seasonal changes are crucial.
Technology plays a pivotal role in optimizing sunlight capture. Remote sensing tools, such as drones equipped with multispectral cameras, can provide detailed maps of sunlight distribution across fields. These maps help identify shaded areas where sunlight capture can be improved. Additionally, modeling tools can simulate various crop configurations and predict their impact on sunlight absorption, allowing farmers to make data-driven decisions.
Incorporating these practical applications and techniques into farming practices not only enhances crop productivity but also contributes to sustainable agricultural development. By leveraging both traditional and technological approaches, farmers can create more efficient and resilient farming systems.
Future Trends and Innovations
As the agricultural sector continually evolves, future trends and innovations in crop configurations and sunlight capture are set to play a pivotal role. Advancements in plant breeding and genetic engineering are at the forefront of these developments. By harnessing the power of biotechnology, researchers are making strides in improving photosynthetic efficiency, allowing crops to convert more sunlight into energy. This not only boosts yield but also enhances the resilience of crops to environmental stresses.
One promising innovation is the engineering of plants with enhanced light-harvesting complexes. These complexes are designed to optimize the absorption of sunlight, ensuring that plants make the most of available light. Additionally, the development of crops with altered leaf structures that can more effectively capture and utilize sunlight is another area of active research. These advancements could revolutionize how crops are grown, making them more efficient and productive.
Climate change poses a significant challenge to agriculture, impacting sunlight availability and crop growth patterns. As global temperatures rise and weather patterns become more erratic, the need for adaptive agricultural practices becomes critical. Innovative solutions such as precision agriculture, which uses data analytics and satellite imagery to optimize crop configurations and manage sunlight capture, are being developed to address these challenges. These technologies enable farmers to make informed decisions, ensuring that crops receive optimal sunlight and other resources.
The broader implications of these advancements for global food security and sustainable agriculture are profound. By improving the efficiency of sunlight capture and adapting to changing climate conditions, these innovations can help ensure a stable food supply for the growing global population. Additionally, sustainable agricultural practices that reduce the environmental impact of farming are essential for preserving natural resources for future generations.
In conclusion, the future of crop configurations and sunlight capture lies in the integration of advanced technologies and innovative practices. As research continues to push the boundaries of what is possible, the agricultural sector must remain adaptable and forward-thinking to meet the challenges of tomorrow.